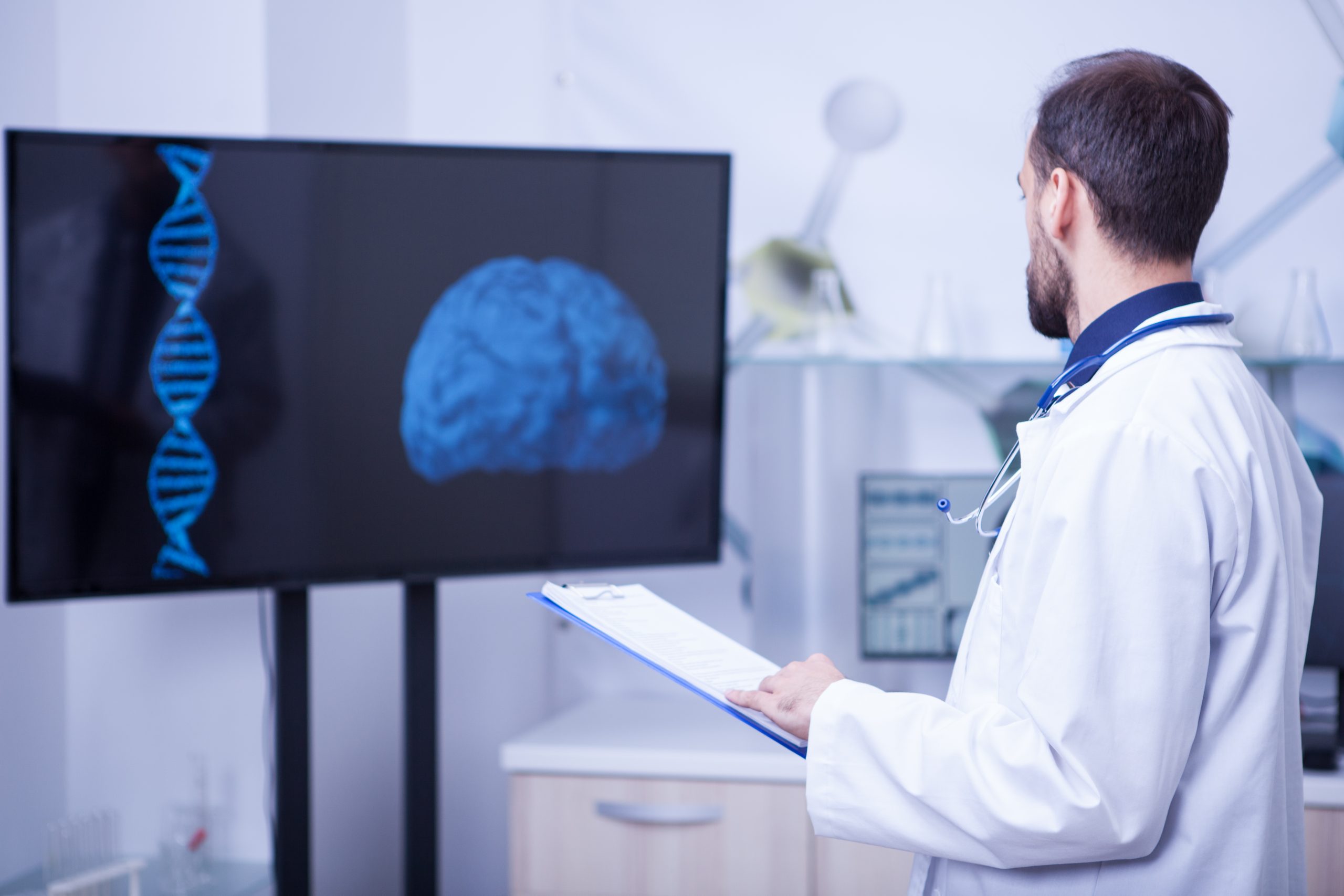
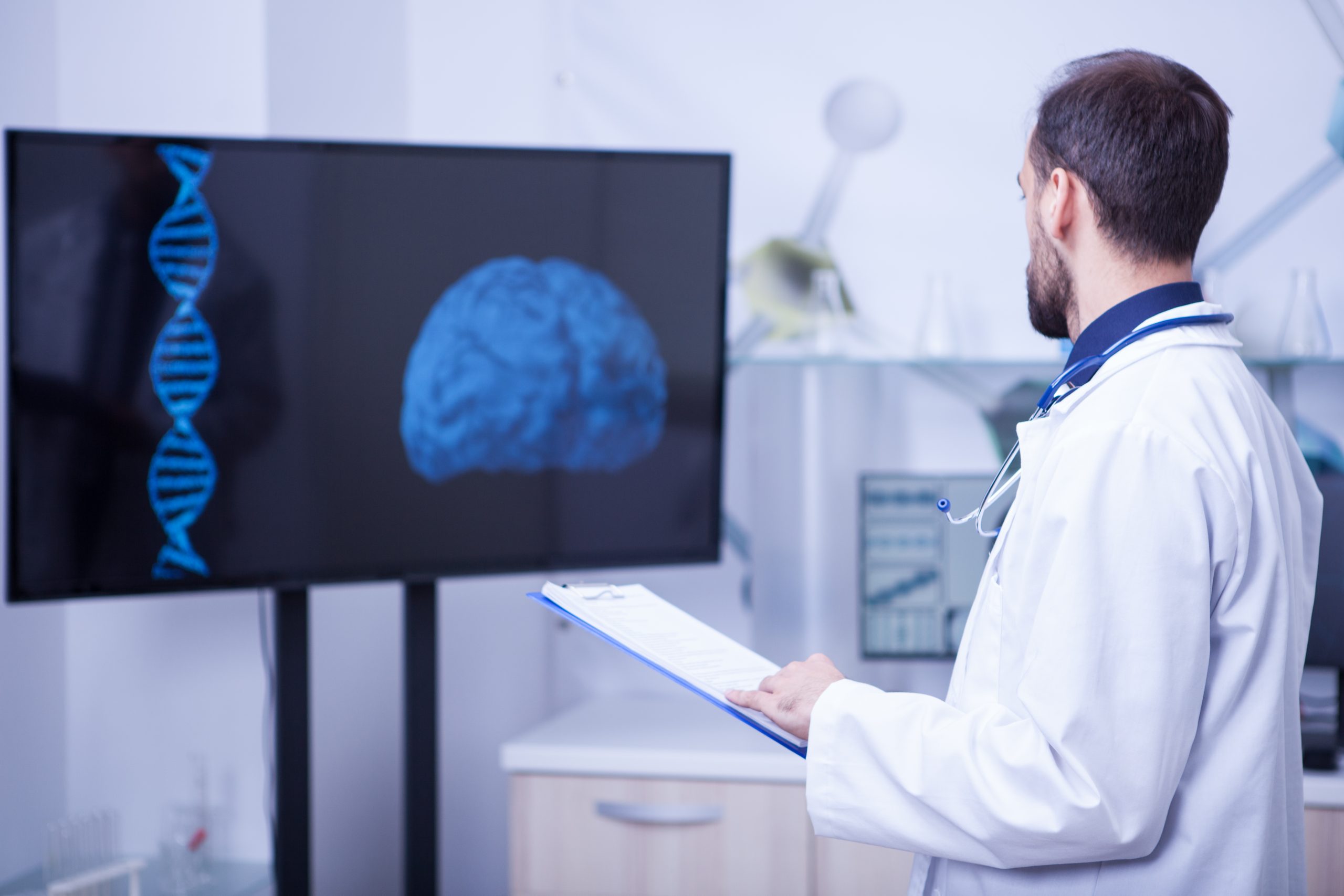
Because mature nerve cells do not rebuild their connections, nerve injuries might induce blindness or paralysis. A team of UConn School of Medicine researchers recently reported in Development that everyone has at least a small number of nerve cells that can be induced to sprout, potentially restoring sight and mobility.
Glaucoma; optic neuritis; optic nerve trauma or stroke: All of these disorders have the potential to permanently damage the optic nerve, resulting in blindness. Glaucoma alone affects almost 3 million people in the United States. According to the Christopher Reeve Foundation, nerve injury that results in paralysis affects around 5 million people in the United States.
Although blindness and paralysis appear to be quite distinct, many forms of these two disorders are caused by the same underlying cause: neurons whose axons, or lengthy fibers that connect the nerve to the brain or spinal cord, are cut and never grow back. Axons function as wires, carrying electrical impulses from different regions of the body to the central nervous system. When a wire is severed, it can no longer carry signals and the link is lost. Similarly, if the axons in the optic nerve are unable to reach the brain or the axons from your toe are unable to attach to the spinal cord, you would be unable to see or move your toe.
Some species, such as mice and humans, may regenerate axons. It was considered that animals lacked the young nerve cells required. However, a team of researchers led by UConn School of Medicine neurologist Ephraim Trakhtenberg discovered differently. They report the discovery of neurons that act similarly to embryonic nerve cells in a publication published on April 24 in Development. The neurons express a similar group of genes and can be experimentally encouraged to rebuild long-distance axons, which could lead to the restoration of some vision disorders caused by nerve damage under the correct conditions.
Furthermore, the researchers discovered that mitochondria-associated Dynlt1a and Lars2 genes were upregulated in these neurons during experimental axon regeneration, and that activating them in injured neurons via gene therapy promoted axon regeneration, identifying these genes as novel therapeutic targets. Trakhtenberg believes that analogous immature nerve cells exist in areas of the brain other than the visual system and that, given the appropriate conditions, they could cure some aspects of paralysis.
However, the right circumstances are tough to come by. When activated by a treatment, the axons of these embryonic-like nerve cells begin to rebuild in wounded locations, although they tend to stall before reaching their original targets. Previous study has found that a combination of cell maturity, gene activity, signaling molecules within axons, as well as scarring and inflammation at the damage site, all appear to limit axon regrowth. Some therapies that target genes, signaling molecules, and the environment of the damage site can induce axons to grow, but they seldom grow long enough.
Trakhtenberg lab researchers began investigating the behavior of another type of cell, oligodendrocytes. If axons are the nervous system’s cables, oligodendrocytes are the insulation. Myelin is a protein that insulates axons and enhances conductivity. It also stops axons from forming superfluous connections, which is critical. Axons in embryos typically grow to their full length before being covered with myelin. However, Trakhtenberg and postdoctoral fellow Agniewszka Lukomska discovered that in certain damage areas, the cells that apply myelin begin interacting with the regenerated axons immediately after they begin sprouting. That contact, which occurs before the insulation process, contributes to axons halting and never reaching their destinations.
This discovery is described in a study published in Development on April 27. To fully restore broken axons, the researchers believe a multi-pronged strategy is required. To stimulate nerve cells to grow like embryonic nerve cells, therapies that target both the gene and signaling activity within them would be required. By removing inhibitory chemicals from the environment and preventing oligodendrocytes from insulating, axons would have more time to reconnect with their targets in the central nervous system before being myelinated. The healing process would then be completed by treatments that induce oligodendrocytes to myelinate the axons.
Although protection by myelination of still intact but demyelinated axons from ensuing inflammatory damage may take precedence in some types of complex injuries, Trakhtenberg believes that secondary inflammatory damage can eventually be controlled pharmacologically, paving the way for pausing myelination and unhindering therapeutic axon regeneration for these types of lesions as well.
The new understanding of how axons form could pave the way for truly effective medicines for blindness, paralysis, and other nerve-related illnesses. But the research has a deeper meaning for Trakhtenberg. It provides solutions to some of the most pressing concerns about how our neural systems grow.
“If you succeed in regenerating injured neural circuits and restoring function, this would indicate that you are on the right track toward understanding how at least some parts of the brain work,” Trakhtenberg says. The researchers are currently working on a deeper understanding of the molecular mechanisms behind both axon growth and interaction with oligodendrocytes.
more recommended stories
New Blood Cancer Model Unveils Drug Resistance
New Lab Model Reveals Gene Mutation.
Healthy Habits Slash Diverticulitis Risk in Half: Clinical Insights
Healthy Habits Slash Diverticulitis Risk in.
Caffeine and SIDS: A New Prevention Theory
For the first time in decades,.
Microbial Metabolites Reveal Health Insights
The human body is not just.
Reelin and Cocaine Addiction: A Breakthrough Study
A groundbreaking study from the University.
Preeclampsia and Stroke Risk: Long-Term Effects
Preeclampsia (PE) – a hypertensive disorder.
Statins and Depression: No Added Benefit
What Are Statins Used For? Statins.
Azithromycin Resistance Rises After Mass Treatment
Mass drug administration (MDA) of azithromycin.
Generative AI in Health Campaigns: A Game-Changer
Mass media campaigns have long been.
Molecular Stress in Aging Neurons Explained
As the population ages, scientists are.
Leave a Comment