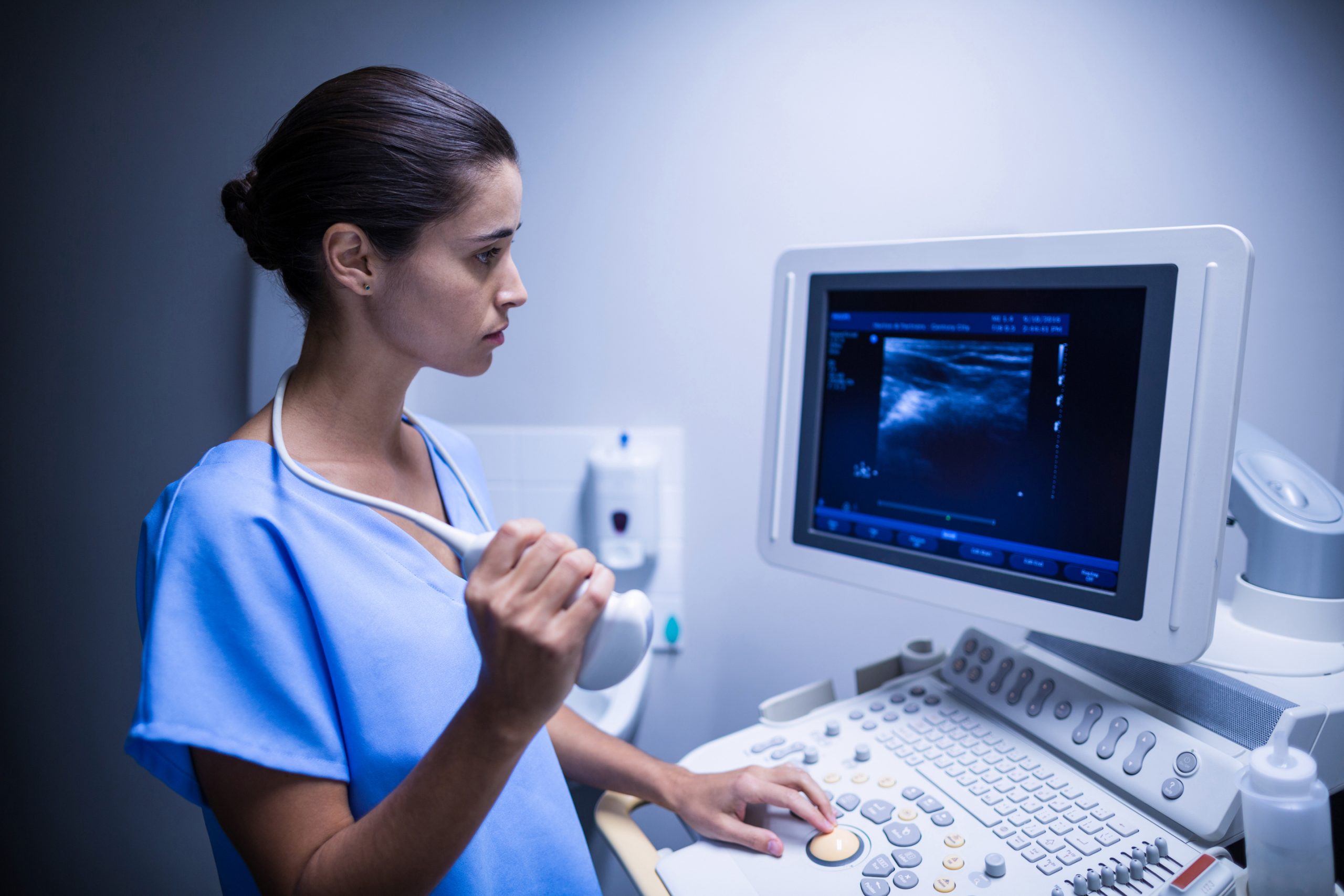
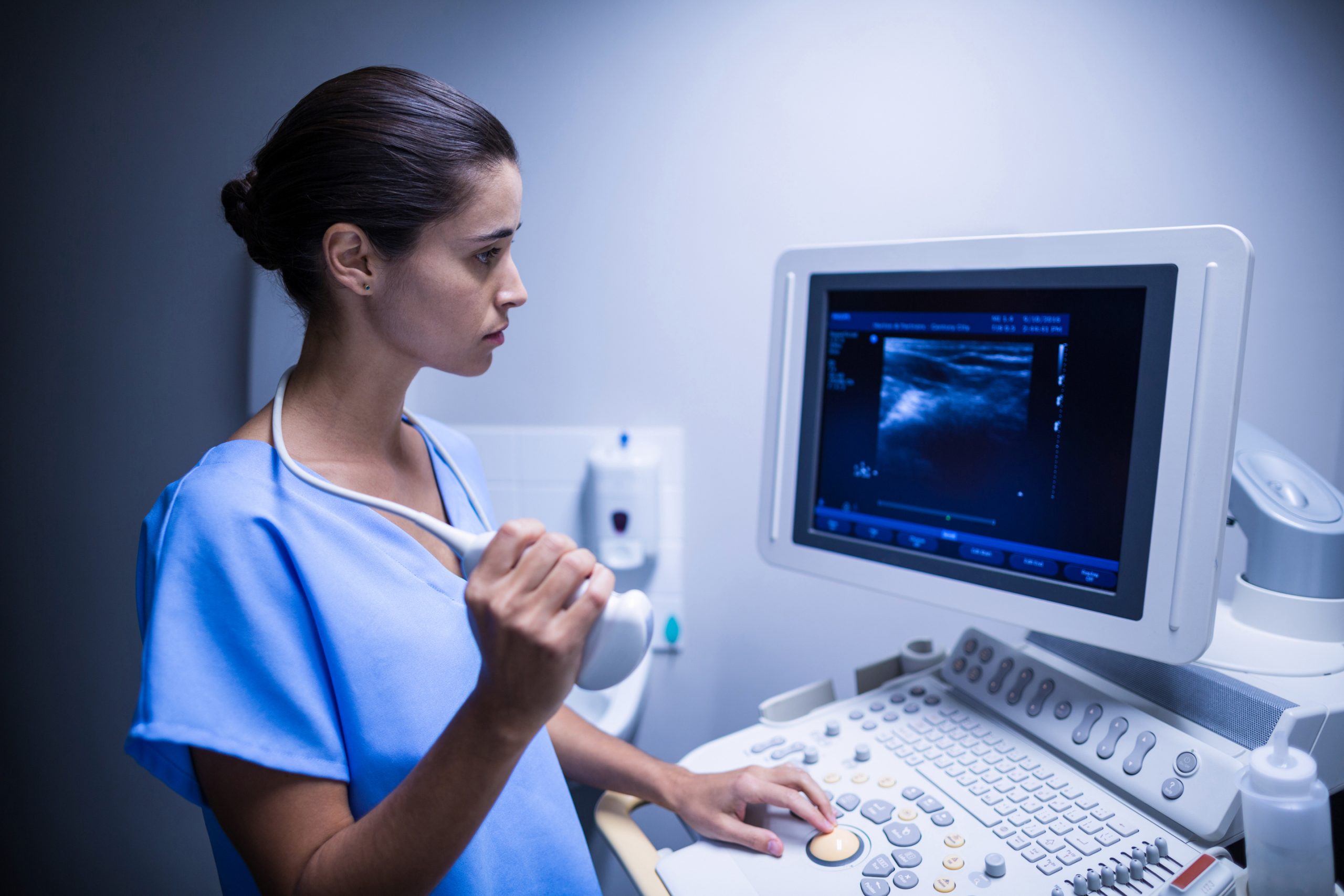
The Noncontact Laser Ultrasound (NCLUS) was created by MIT Lincoln Laboratory researchers and collaborators at the Massachusetts General Hospital (MGH) Center for Ultrasound Research and Translation (CURT). This laser-based ultrasound technology produces images of inside organs, fat, muscle, tendons, and blood arteries. The technology also assesses bone strength and may be able to detect disease progression over time.
“Our patented skin-safe laser system concept seeks to transform medical ultrasound by overcoming the limitations associated with traditional contact probes,” says principle investigator Robert Haupt, a senior staff member in the Active Optical Systems Group at Lincoln Laboratory. The system was co-invented by Haupt and senior staff member Charles Wynn, with assistant group leader Matthew Stowe providing technical leadership and control of the NCLUS initiative. Rajan Gurjar leads the system integrator team, with Jamie Shaw, Bert Green, Brian Boitnott (currently at Stanford University), and Jake Jacobsen working on optical and mechanical engineering and system development.
Medical ultrasonography in use
If your doctor orders an ultrasound, a highly experienced sonographer will push and control an array of transducers mounted in a handheld device onto your body. High-frequency acoustic waves (ultrasound waves) enter and propagate through your bodily tissue as the sonographer moves the transducer probe across your skin, where they “echo” off distinct tissue structures and features. These echoes are caused by changes in tissue strength (tissue softness or stiffness) from fat, muscle, organs, blood vessels, and bone deep within the body. The returning echoes are received by the probe and combined into representational pictures of the body’s interior features. Specialized processing algorithms (synthetic aperture processing) are utilized to generate the forms of the tissue features in 2D or 3D, which are subsequently presented in real time on a computer monitor.
Doctors can use ultrasound to noninvasively “see” within the body and scan various tissues and their geometries. Ultrasound can also be used to quantify blood flow flowing through arteries and veins, as well as define the mechanical properties of tissues and organs (elastography). Ultrasound is commonly used to help clinicians evaluate and diagnose a wide range of health issues, diseases, and accidents. Ultrasound can, for example, be used to examine the anatomy of a developing fetus, diagnose malignancies, and assess the degree of constriction or leakage in heart valves. Ultrasound is very portable, relatively inexpensive, and frequently employed in point-of-care and remote-field settings, ranging from handheld devices on an iPhone to cart-based systems.
Ultrasound’s Limitations
Despite the fact that cutting-edge medical ultrasound equipment can resolve tissue characteristics to fractions of a millimeter, the technology has several limits. Sonographers’ freehand movement of the probe to acquire the optimal viewing window into the body interior results in imaging mistakes. More specifically, when sonographers apply pressure to the probe by feel, they arbitrarily compress the local tissue with which the probe comes into touch, resulting in unanticipated changes in tissue properties that affect the travel routes of the ultrasonic waves. This compression alters tissue-feature images with some randomness, resulting in inaccurate plotting of feature shapes. Furthermore, even a tiny tilt of the probe affects the angle plane of the image view, skewing the image and producing doubt about where features are located in the body.
The picture distortion and positional reference uncertainty are high enough that ultrasound cannot reliably determine, for example, whether a tumor is growing or shrinking and precisely where the tumor is located in the host tissue. Furthermore, even if the same sonographer is retracing their steps, the uncertainty in feature size, shape, and position will fluctuate with each repeat measurement. This uncertainty, known as operator variability, is exacerbated when multiple sonographers try the same measurement, resulting in inter-operator variability. Because of these limitations, ultrasonography is frequently used to track malignant tumors and other disease conditions. Instead, technologies such as magnetic resonance imaging (MRI) and computed tomography (CT) are required to track illness progression, despite their significantly higher cost, increased system size and complexity, and radiation danger.
“Variability has been a major limitation of medical ultrasound for decades,” says Anthony Samir, MGH Radiology associate chair of Imaging Sciences and director of CURT. Samir and his MGH CURT colleagues Kai Thomenius and Marko Jakolvejic give the laboratory team with crucial medical knowledge, technical understanding, and direction on traditional ultrasound devices, as well as participate on NCLUS system development.
NCLUS has the potential to decrease the requirement for a sonographer while also mitigating operator variability by entirely automating the process of capturing ultrasound pictures. The laser placement may be precisely duplicated, removing fluctuation between repeated observations. Because the measurement is noncontact, no localized tissue compaction or image feature distortion occurs. Furthermore, like MRI and CT, NCLUS has a fixed-reference-frame capacity that uses skin markers to recreate and compare repeat scans throughout time. The laboratory team created software that analyses ultrasound images and detects differences between them to provide such tracking capabilities. NCLUS is also appropriate for patients with painful or sensitive body parts, in delicate conditions, or at risk of infection because it does not require physical pressure or coupling gels (as do contact probes).
NCLUS could image burn or trauma victims, patients with open deep-tissue regions directly during surgery, premature infants requiring intensive medical care, patients with neck and spine injuries, and contagious individuals from standoff distances,” Haupt says.
Ultrasound waves caused by light
NCLUS uses a pulsed laser to deliver optical energy through the air to the skin’s surface, where it is rapidly absorbed. The optical pulse causes instantaneous localized heating and quick skin deformation via a thermoelastic process, which generates ultrasonic waves and acts as an ultrasound source – a phenomena known as photoacoustics.
The optical pulse generates enough ultrasound strength at frequencies comparable to those used in medical ultrasound while causing no skin sensation. The team patented the optical carrier wavelength selection, with the photoacoustic technique designed to produce a consistent ultrasonic source regardless of skin color or tissue roughness.
The ultrasound echoes that return from the tissue interior appear as localized vibrations at the skin’s surface, which are detected by a very sensitive, specialized laser Doppler vibrometer.
“With an appropriate laser transmit-and-receive implementation, any exposed tissue surfaces can become viable ultrasound sources and detectors,” Haupt explains.
Progress toward a therapeutically operable system
The researchers demonstrated in 2019 that the NCLUS proof-of-concept (GEN-1) system can gather ultrasound imaging from human subjects using skin-safe lasers, a medical first. However, the time required to collect image data from the patient subject was lengthy and impractical for clinical use. Furthermore, the image resolution of the GEN-1 system was much lower than that of state-of-the-art medical ultrasound.
Since then, significant engineering progress has occurred in order to transfer NCLUS GEN-1 to an operational system suitable for clinical testing. Both the laser source and receiver in the clinical NCLUS system are downsized and contained inside an optical head linked to a portable armature. The lasers that pulse and scan are 500 times quicker than those used in the GEN-1 system, resulting in an image-data acquisition period of less than a minute. Future NCLUS prototypes will have acquisition times that are less than one second. The new clinical system also uses far higher ultrasound frequencies than the GEN-1 system, allowing for resolution of up to 200 microns, which is comparable to the resolution of state-of-the-art medical ultrasonography.
The adjustable armature provides numerous degrees of flexibility for viewing various bodily regions. There are additionally programmable fast-steering mirrors inside the optical head that automatically position the source and receive laser beams to precisely build the ultrasonic array. A 2D lidar is utilized to map the patient’s skin surface topography, while a high-frame-rate short-wave-infrared camera captures the laser source and receiver projected locations on the skin, providing the array parameters needed to create ultrasound pictures. Natural skin characteristics such as freckles are used to register skin-surface topography mapping and laser-position recordings. This creates a fixed reference frame for performing exact repeat scans throughout time.
The NCLUS clinical system uses synthetic aperture processing to provide completely automated and registered ultrasound pictures. The technology was shown on a gel-based puck produced to mimic the mechanical qualities of human flesh (referred to as a phantom) that influence the propagation of ultrasonic waves.
The team is now building NCLUS to serve field-forward military applications through sponsored programs. These applications include detecting and characterizing life-threatening injuries caused by internal organ bleeding, monitoring debilitating musculoskeletal injuries and their healing over time, and providing elastographic imagery of amputee limb regions to speed up the design and fitting of prosthetic sockets. Imaging in the intensive care unit is one example of a civilian application. With NCLUS, emergency medical technicians, paramedics, and medical personnel who do not have specialist sonography training may be able to provide ultrasound imaging outside of a hospital – in a doctor’s office, at home, or on a remote battlefield.
“With further development, NCLUS has the potential to be a transformative technology: an automated, portable ultrasound platform with a fixed-reference-frame capability similar to that of MRI and CT,” Samir says.
The researchers will conduct clinical trials utilizing an operational skin-safe laser to examine ultrasound images and compare them to those of traditional medical ultrasonography in the next phase of the NCLUS initiative. If these tests are successful, the researchers will seek commercial funding for clinical medical device development, which will be followed by FDA agency approval.
The US Army Military Operational Medicine Research Program is funding this research. The MIT Committee on the Use of Humans as Experimental Subjects approved the human in vivo testing.
more recommended stories
Anxiety’s Impact on Dementia
Researchers evaluated the longitudinal association between.
Overcoming Cancer Relapse with CAR-Enhancer Therapy
CAR T-cell therapies have transformed the.
Massive Neuroimaging Dataset Boosts Stroke Research
Using large-scale neuroimaging can help advance.
Genetic Variants & Disease Risk Insights
The relationship between DNA methylation and.
Shingles Vaccine Lowers Dementia Risk
A recent study published in Nature.
Game of Thrones Advances Face Blindness Research
Relationship building can be difficult for.
Gut Microbes Linked to Alzheimer’s Disease
Researchers examined the available information on.
FDA Alternatives to Big Pharma Weight Loss Drugs
Mark Mikhael, a pharmacist, has shed.
Blood Test for Early Parkinson’s Detection and Treatment
Parkinson’s disease (PD) is currently diagnosed.
Beyond Anti-Obesity: Glucagon-Like Peptide-1 Benefits
Researchers examined clinical data on the.
Leave a Comment